Diamond Wafers: Production Technologies and Applications
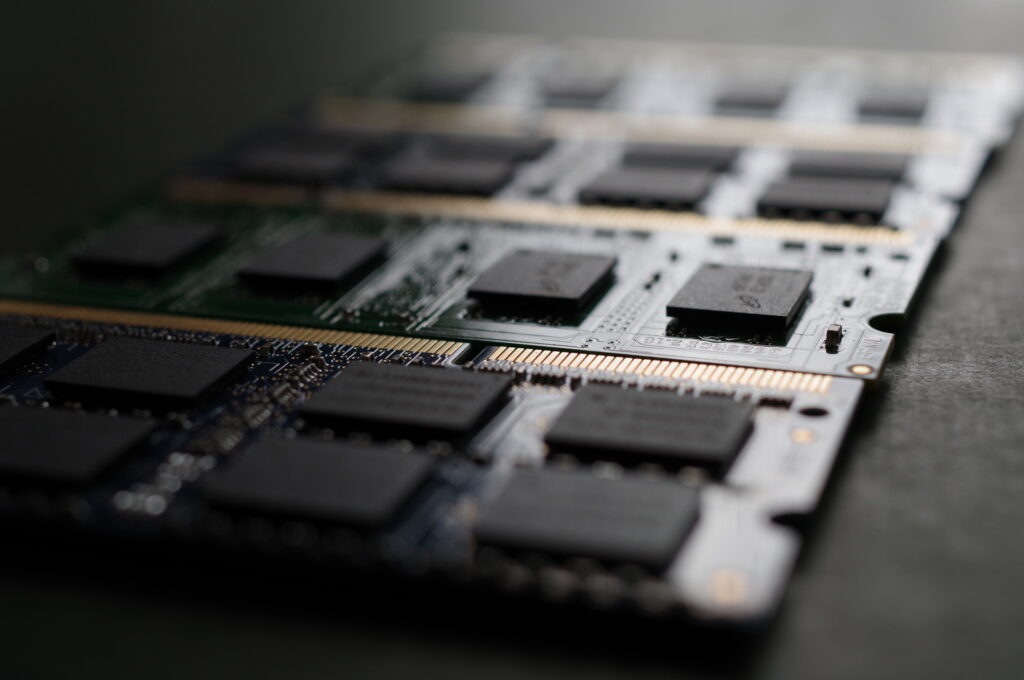
The properties of diamond are ideal for power devices and devices operating in extreme environments. Although diamond’s practical use has been limited by challenges in mass production, this situation continues to evolve, as numerous research institutions, including Orbray, have made significant advancements in diamond wafer production technology.
This article presents production technologies for diamond semiconductor wafers and explores potential future applications of diamond semiconductors.
Table of contents [close]
- 1. The Ideal Properties of Diamond Semiconductors
- 2. Applicable Fields
- 3. The Challenge of Producing Large-Diameter Diamond Wafers
- 4. Epitaxial Growth
- 5. Heteroepitaxial Growth
- 6. Orbray’s Crystal Growth Technology
- 7. Chemical Mechanical Polishing
- 8. Summary|High-quality diamond semiconductors require crystal growth and polishing technology
The Ideal Properties of Diamond Semiconductors
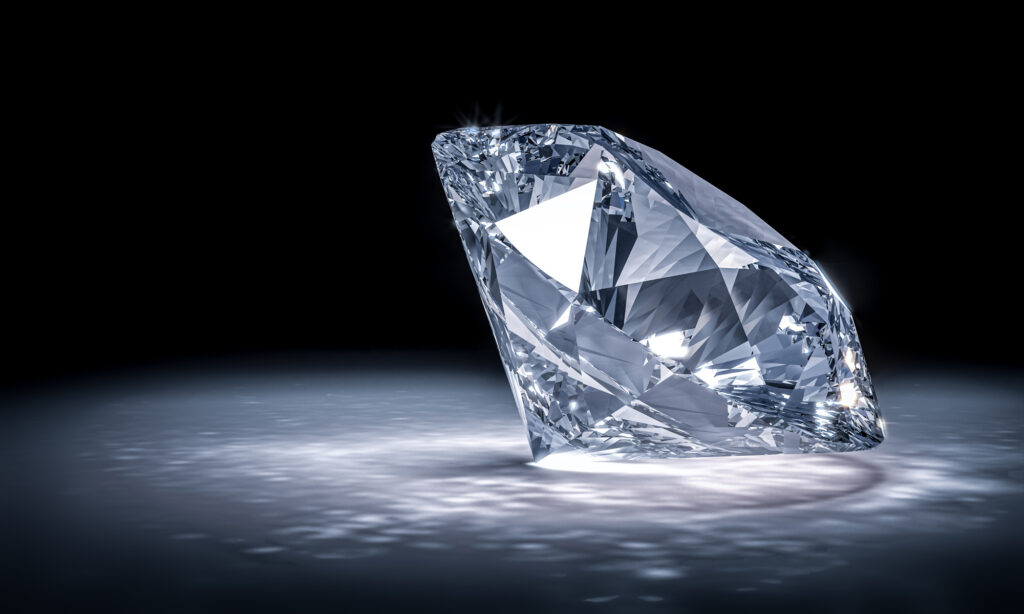
Diamond is often referred to as the “ultimate power semiconductor material” due to its exceptional physical properties. Some of these include:
・A large band gap, leading to high resistance to dialectic breakdown.
・High mobility (switching speed), resulting in low power loss.
・Operational stability in high-temperature and high-radiation environments.
For more details on the band gap and mobility, please refer to the article “Power Semiconductors”.
Applicable Fields
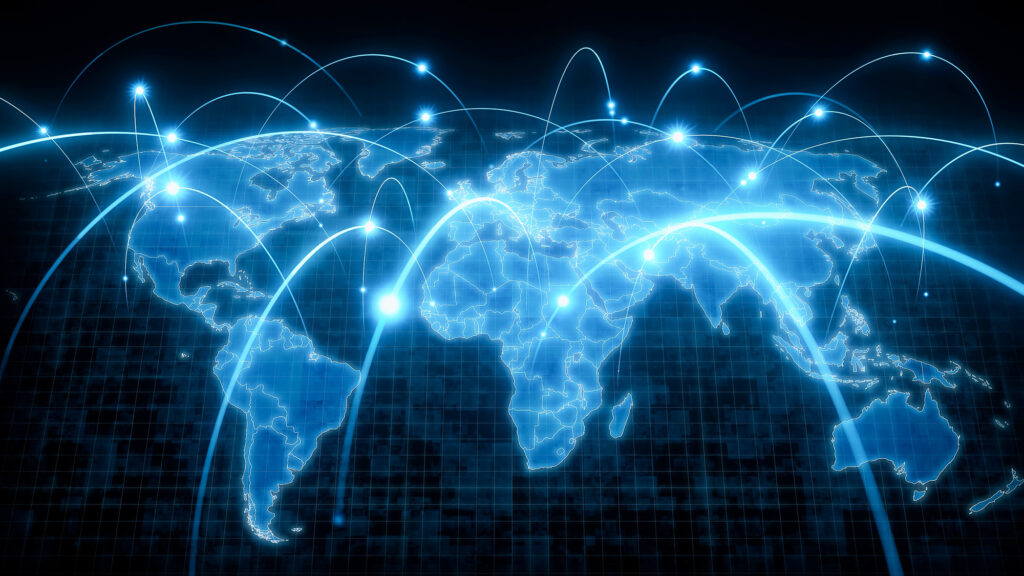
Owing to its unique properties, diamond semiconductors are expected to become important components in control devices for equipment operating in extreme environments, including power devices.
Communications Satellites
One potential application field for diamond semiconductors is communications satellites.
Communications satellites, which communicate with the ground via radio waves, require high frequencies and power to operate effectively. The early communications satellites used vacuum tubes. However, vacuum tubes are inefficient due to their large size and significant energy losses. Although vacuum tubes have been expected to be replaced with semiconductors, the harsh environment of outer space can interfere with their operation.
Diamond semiconductors offer a potential solution, as they are compact and capable of withstanding the frequencies and outputs required by communication satellites, enabling highly efficient signal amplification. They are also resistant to heat and cosmic rays, allowing for stable operation.
Nippon Telegraph and Telephone Corporation (NTT), an owner of communication satellites, has been a frontrunner in conducting research in this field. Orbray is also engaging in long-term exposure experiments on the International Space Station, and making progress towards practical application of this technology.
Radiation Sensors
The Great East Japan Earthquake in March 2011 and the resulting tsunami caused massive damage at the Tokyo Electric Power Company's Fukushima Daiichi Nuclear Power Plant, resulting in a leak of radioactive material. Due to radiation exposure, sensors throughout the plant quickly deteriorated, and electronic equipment malfunctioned, hindering efforts to assess and address the situation, and preventing the decontamination and dismantling necessary for decommissioning of the facility.
In response to these challenges, Okuma Diamond Device, established in 2022 through a collaboration between Hokkaido University and Japan’s National Institute of Advanced Industrial Science and Technology (AIST), is making technological progress towards using diamond semiconductors in nuclear decommissioning.
Diamond semiconductor devices are capable of operating at high temperatures (up to ~300℃) and in strong radiation environments (up to ~300 MGy), making them suitable for use in harsh conditions.
Quantum Computing
Quantum computers can perform certain calculations significantly faster than conventional computers. Japan’s Institute of Physical and Chemical Research (RIKEN) and others have succeeded in developing quantum computers, but the current models are unstable and only function on a small scale. One major challenge hindering their practical application is that qubits (quantum bits) have been difficult to precisely control.
Qubits typically face issues like an inability to maintain quantum states for extended periods of time, and difficulties in access. To address this, a research group led by Professor Kosaka at Yokohama National University is working to create easily controllable qubits using diamond.
They are utilizing a lattice defect in diamonds known as the diamond nitrogen-vacancy (NV) center as a qubit. By replacing carbon atoms in diamond with nitrogen atoms, lattice defects are created. These defects are known to capture spins that maintain their quantum states for long durations.
The research group has developed a novel method called the optically addressed quantum gate, where light is used to select the NV center to be manipulated, and quantum control is executed using highly reliable microwaves and radio waves. They have experimentally demonstrated the ability to control spin with high spatial resolution and fidelity.
The Challenge of Producing Large-Diameter Diamond Wafers
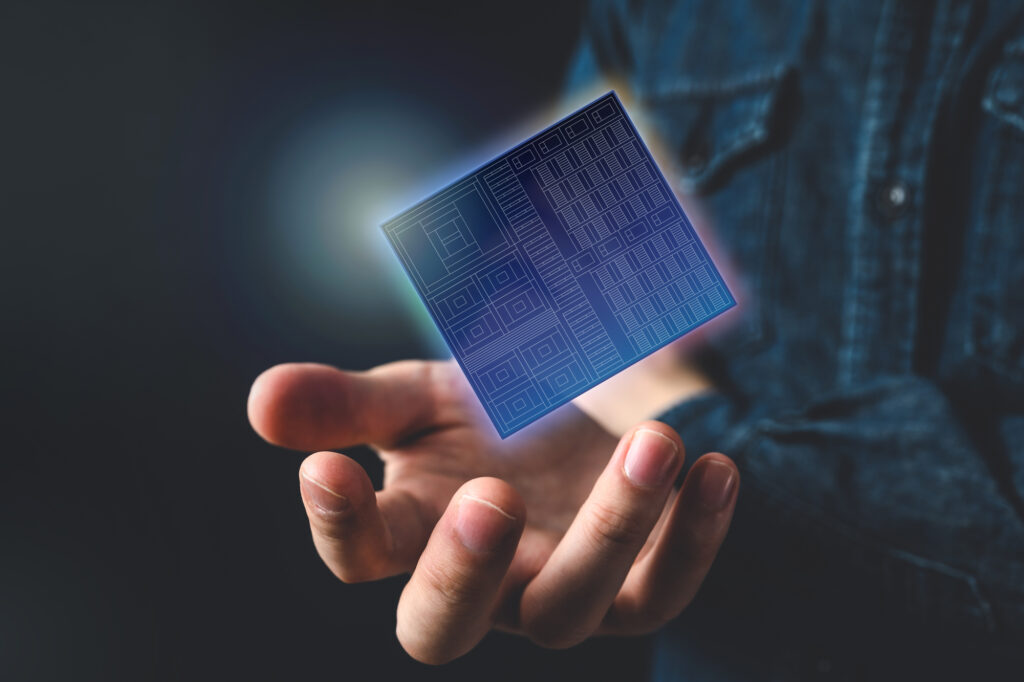
Until now, artificial diamond synthesized under high-temperature and high-pressure environments has been used for research purposes. High-quality diamond suitable for semiconductor applications can be created by slowly synthesizing carbon over a period of a week or more.
However, for practical use in the market, a large quantity of diamond semiconductor devices will be needed. This demands diamond wafers larger than those currently available.
Epitaxial Growth
The manufacture of large diamond wafers relies on a technique for growing crystals known as epitaxial growth. This process involves growing the target crystal on top of a substrate crystal.
One way of achieving epitaxial growth is the chemical vapor deposition (CVD) method, in which a mix of raw material gases is directed onto the surface of a substrate to facilitate growth of the target material. This method is used in the production of GaN thin films.
Among the CVD methods, diamonds can be synthesized using the plasma CVD method, which utilizes ionized gas.
Heteroepitaxial Growth
Epitaxial growth is divided into two types, based on the substrate material used. Homoepitaxial growth occurs when the substrate and the thin film grown on it are made of the same material. If different materials are used, this is called heteroepitaxial growth.
Creating a large-diameter wafer necessitates a large-diameter substrate. For diamonds, preparing a large-diameter diamond crystal to be used as the base substrate is difficult, so homoepitaxial growth for making large-diameter diamond wafers is unfeasible. On the other hand, in heteroepitaxial growth, if the underlying substrate is large, diamonds of the same size can theoretically be produced.
A major challenge in heteroepitaxial growth is achieving compatibility between the substrate crystal and the film grown on top of it. The growth of the crystal is heavily influenced by the underlying substrate crystal. Simply exposing the surface to the crystal precursors can cause significant distortion, preventing the formation of a clean crystal.
This distortion was once a problem in GaN crystal growth as well. Sapphire substrates, which have a lattice constant markedly different from GaN, are used for heteroepitaxial growth of GaN. The late Nobel Prize laureate Dr. Isamu Akasaki (1929-2021), of Meijo University and Nagoya University, managed to successfully grow GaN heteroepitaxially by introducing a buffer layer atop the substrate to mitigate strain in the wafer.
Similarly, in heteroepitaxial growth of diamond, distortions must be avoided. Moreover, materials shrink when cooled, with each substance having its own coefficient of thermal expansion. This difference in thermal expansion coefficients between the diamond and the underlying substrate can also cause distortion.
Orbray’s Crystal Growth Technology
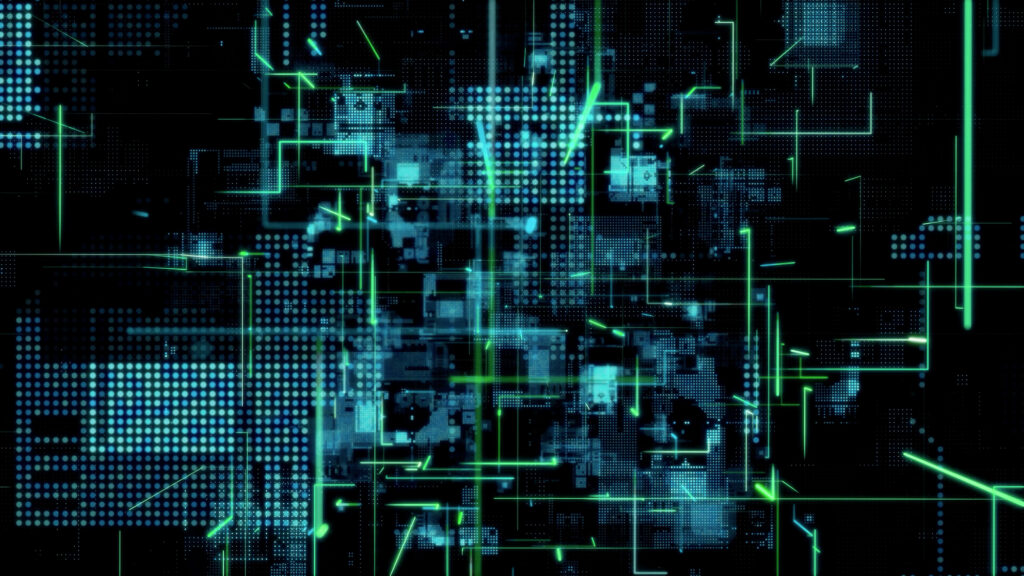
Orbray has dedicated many years to researching and developing technology for producing high-purity, large-diameter diamond wafers.
In September 2014, we filed a patent for a diamond manufacturing technology known as the microneedle method. This technique involves forming numerous high aspect diamond pillars on an iridium/sapphire substrate and growing the diamond crystal on top of these pillars to create a large, unified wafer. Using this method, we successfully fabricated a 1-inch diameter diamond wafer.
However, this microneedle method is complex and costly. To address this, in 2021, Orbray developed the step-flow method for growing diamond crystals.
The step-flow method involves growing crystals on a sapphire base layer whose surface is slightly tilted against the crystal structure, creating “steps” on the surface. This method of growing diamond crystals reduces stress in the diamond thin film, resulting in a wafer with fewer defects. We were able to successfully produce a 2-inch diamond wafer and reduce costs compared to previous methods.
For more information, Step-flow growth and Microneedle growth
Chemical Mechanical Polishing
A process called chemical mechanical polishing (CMP) has been established in the industry in the fabrication of devices on semiconductor substrates like silicon. The method involves first smoothing the wafer surface through polishing, and then finishing it with colloidal silica to attain a perfectly smooth surface at the atomic level. However, because diamond is the hardest and most inert material known, conventional surface polishing methods cannot be used to polish diamond wafers. Through our many years of processing high-precision jewels, including diamonds, Orbray has developed diamond processing technologies that can achieve atomic-level smooth surfaces. Furthermore, since traditional CMP methods are unsuitable for diamond, we have innovated our own unique approach.
In CMP, the object's surface is polished while simultaneously being dissolved or softened by chemicals contained in the polishing slurry.
For additional information about CMP, please visit (https://orbray.com/magazine_en/archives/1313).
Summary|High-quality diamond semiconductors require crystal growth and polishing technology
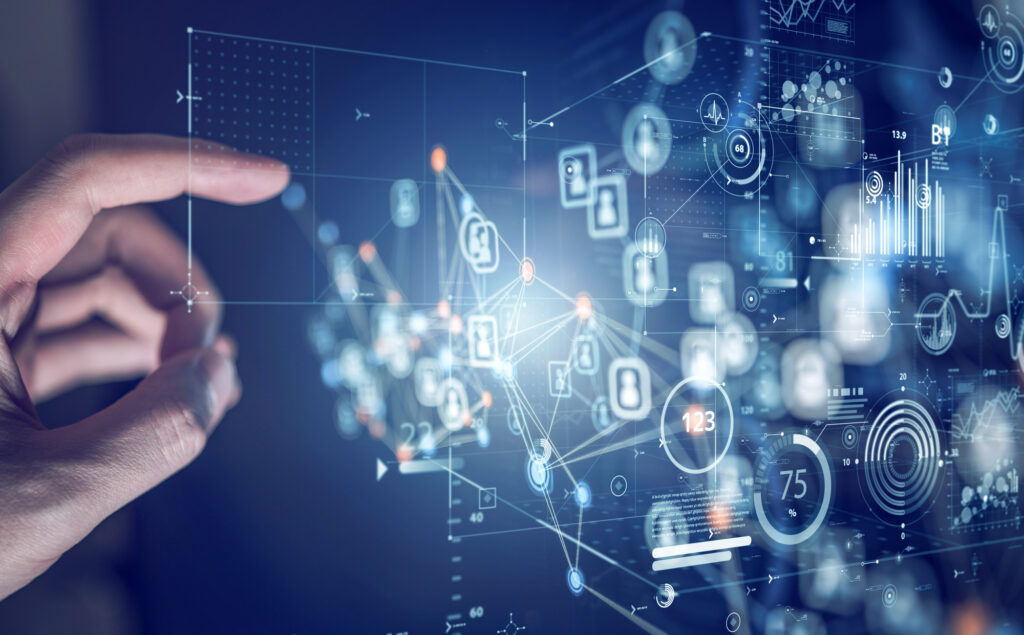
Diamond semiconductors are expected to play important roles in a variety of applications in the future, such as communications satellites, nuclear power plant decommissioning equipment, and quantum computers. But many difficulties in increasing the quality and size of diamond wafers remain. Orbray has continued to refine essential technologies for producing high-quality diamond wafers over the years. Through these persistent efforts, Orbray is on the verge of commercializing this technology. We remain committed to advancing the production of diamond semiconductor wafers and contributing to growth and innovation in the field.
-
Introduction to suction nozzles and collets
-
Surface polishing technologies - Essential technologies for semiconductor, MEMS, and LED manufacturing
-
Jewel bearings: Orbray and the watch industry [Story of Watch 1]
-
Space Delivery Project: Return to Earth
Initial shipment launched
Scheduled to return to Earth
after 6 months of exposure to outer space -
Orbray Wins Second Consecutive “Semiconductor of the Year” Award in 2023
-
We were featured on the cover of the journal Diamond and Related Materials.