The role of high-precision processing technology in the development of quantum computing
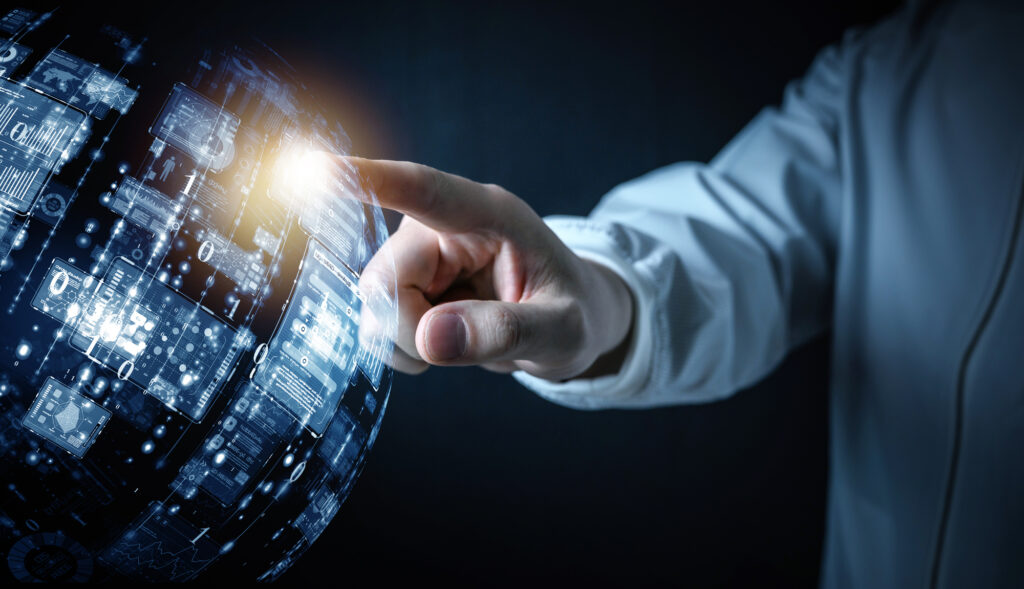
Analysis of complex phenomena such as weather forecasting and data analysis using AI require ultra-fast calculations of vast quantities of information. Conventional computers that use 0s and 1s to perform these calculations will soon reach their performance limits. This had led to growing interest in quantum computers.
In 2019, NTT introduced the Innovative Optical and Wireless Network (IOWN) concept. IOWN will use photoelectronic convergence technology to enhance computational processing speeds and utilize an All Photonics Network (APN), which connects everything at high speed using light signals. In addition, research is being conducted on quantum computers as a completely new computing platform for high-speed data processing.
This article will cover quantum computers, the IOWN concept, and the production and processing technologies that are paving the way for the practical use of quantum computing.
Table of contents [close]
- 1. Quantum computers: Breaking through the limits of semiconductor technology
- 2. Quantum computing: The foundation of the IOWN concept
- 3. Challenges for widespread adoption of quantum computers
- 4. High-speed optical networks are needed to take full advantage of the processing power of quantum computers
- 5. Bringing quantum computers closer to practical use
Quantum computers: Breaking through the limits of semiconductor technology
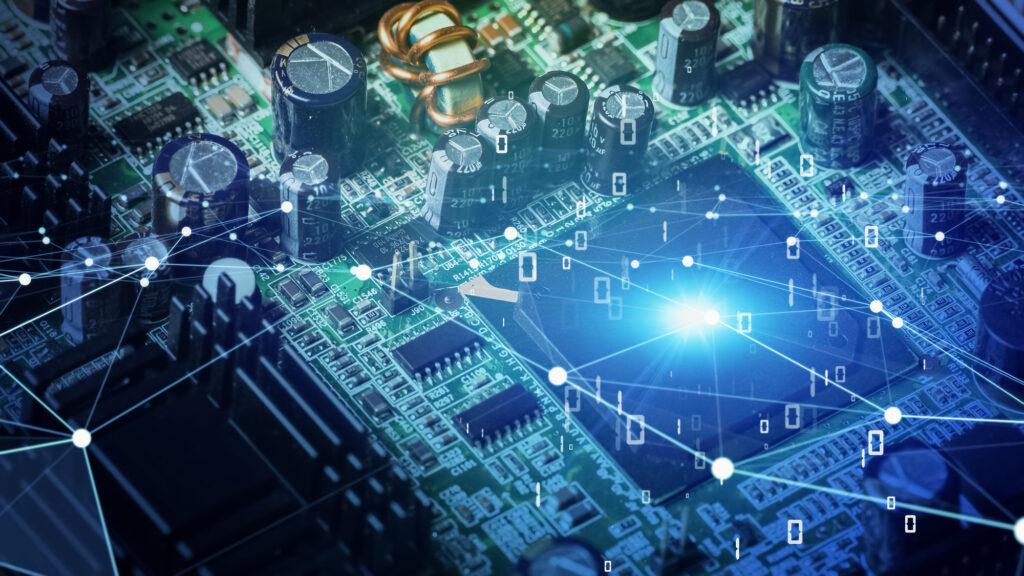
Quantum computers represent the next generation of computing technology, built on the principles of quantum mechanics. Traditional semiconductor-based computers perform calculations by representing data as an electrical signal that is either in the on state (1) or the off state (0). This allows only one piece of information to be processed at one time. The smallest unit of data in this binary system is called a bit.
The smallest unit of information used in quantum computers is the “qubit.” In quantum computers, data is represented using the quantum phenomena of “superposition” and “quantum entanglement.” Superposition is a phenomenon in which two or more opposing states can coexist. Quantum entanglement is a phenomenon in which two or more quantum bits are deeply connected, causing one state to affect the other.
For example, in conventional computers, a single bit can only have either a 0 or 1 state, whereas in quantum computers, superposition allows a quantum bit to have both a 0 and a 1 state at the same time. Furthermore, different quantum bits can be connected and affect each other due to quantum entanglement. As a result, quantum bits can have multiple states at the same time, so they can hold more information than conventional computers, and parallel processing can significantly reduce the processing time for a specific problem. It is expected to bring about great advances in fields such as weather forecasting, logistics forecasting, drug discovery, materials development, and finance.
Quantum computing: The foundation of the IOWN concept
NTT has introduced the IOWN (Innovative Optical and Wireless Network) concept, designed to create a cutting-edge network that offers high capacity, low latency, and low power consumption by moving from traditional electronics to photonics technology. The IOWN framework is built on three core components. The first is the "All Photonics Network (APN)," which integrates photonics-based technology throughout the entire network. The second is the "Cognitive Foundation (CF)," which links all ICT resources to enable flexible and efficient control. The third component, "Digital Twin Computing (DTC)," advances the digital twin concept by replicating the interactions between objects and people in cyberspace.
DTC necessitates a computing board capable of processing data at speeds far beyond conventional systems. Quantum computers are being explored to meet this task. NTT has announced ongoing research and development efforts in both hardware and software to make practical quantum computing a reality.
Challenges for widespread adoption of quantum computers
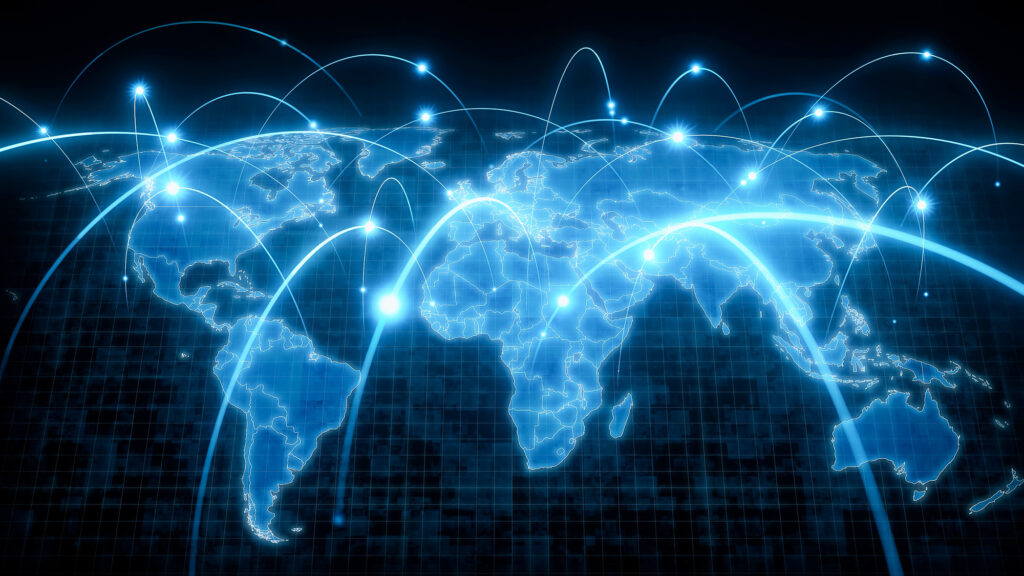
The development of practical quantum computers is currently being pursued by numerous countries and research institutions, including NTT. Despite these efforts, many challenges remain, and experts predict that it will be some time before quantum computers are ready for widespread use.
At present, quantum computers only have around 100 quantum bits, and the computations are prone to errors. While traditional computers use error correction technology, achieving a similar level of error correction in quantum systems is a formidable challenge, requiring over one million quantum bits. Although the integration density of quantum computers continues to improve each year, significantly boosting the number of quantum bits, widespread adoption of this technology will require more development.
Several approaches are being explored to make quantum computers more mainstream. Methods based on superconductivity, light, silicon, ion traps, and cold atoms are being explored. Superconducting quantum computers, which are currently a major focus of research, require ultra-cold temperatures near absolute zero (-273 degrees Celsius) to maintain quantum stability. Scaling up quantum computers to increase the number of quantum bits would necessitate building large-scale cryogenic systems, posing a significant hurdle to practical implementation.
Recently, quantum computers using light have gained attention. These systems use photons—the fundamental particles of light—as quantum bits. Optical quantum computers are advantageous because their quantum bits can function at room temperature, simplifying scaling efforts. They are also compatible with existing photonic technologies, like optical communications devices. Nevertheless, substantial challenges must be addressed before they can be deployed at scale, such as developing reliable light sources for generating optical pulses and enhancing the precision of optical circuit components.
High-speed optical networks are needed to take full advantage of the processing power of quantum computers
In the IWON project, photonic quantum computers, known for their compatibility with All Photonics Networks (APNs), are emerging as a promising candidate for the next generation of computing platforms. Core technologies essential to these systems, such as development of light source modules, have already been developed. If photonic quantum computers are realized, APNs able to transmit large amounts of data at high speeds will become a crucial part of this infrastructure.
Creating APNs requires advanced technologies such as "electrophotonics convergence" and "silicon photonics." Electrophotonics convergence combines electronic circuits, which handle electrical signals, with photonics circuits, which manage optical signals, to enable fast processing with low power consumption. Meanwhile, silicon photonics integrates optical circuits, such as photonics devices like optical waveguides, optical switches, and photoreceivers, and electronic circuits onto a single silicon substrate. This integration paves the way for compact, ultra-fast, large-capacity, low-power communication devices.
Effective utilization of these high-performance communications devices requires precise handling of optical signals received from external systems. To ensure accurate guidance of these optical signals, highly precise connection devices are essential. These specialized components, crafted using advanced processing technologies, enable accurate positioning of the components and minimize signal loss. Examples of such connection devices include:
・Narrow-pitch fiber array
Narrow-pitch fiber arrays are connection devices manufactured using fiber etching technology and V-groove substrate processing technology. Optical fibers are aligned or inserted and fixed in a narrow-pitch V-groove substrate or microhole array, and the end faces are polished to a high flatness of submicron order. They are used when inputting signals from multiple optical fibers to an optical waveguide element, or when splitting signals from an optical waveguide element to multiple optical fibers.
・Bent fiber array
Bent fiber arrays are an innovative way of bending optical fibers with the heat being discharged by the fiber in order to connect the fibers to the array. This innovative method allows optical devices to be made smaller, especially in connections to diffraction grating-type silicon photonics devices.
・Glass capillary
Glass capillaries are crucial for connecting to silicon photonics devices. They offer excellent processability and flexibility compared to other materials, providing high performance in the PC connections of optical connectors. An additional benefit of glass is its high UV light transmittance, which enables rapid and secure fixing of optical fibers, lenses, and other components using ultraviolet-curing adhesives.
・TEC Fusion Technology
TEC fusion technology uses discharge heat within a fusion device to seamlessly connect optical fibers. This technique is essential in manufacturing optical I/O devices, facilitating connections between different diameter MDFs and the waveguides of optical circuits, such as PLC and silicon photonics, as well as standard fibers. TEC fusion enables fibers with varying numerical apertures (NA), cladding diameters, and core diameters, to be connected together, contributing to the versatility and adaptability of fiber connections.
・Collimators and Focusers
Collimators and focusers are optical components designed to either convert light into parallel beams (collimated light) or focus it into a convergent beam. This is achieved using spherical or aspherical lenses attached to the fiber tip, or by precisely processing the fiber tip itself. These components are crucial for directing light efficiently in a specified direction or range and for enhancing the detection efficiency of light that enters a detector from a particular angle.
Bringing quantum computers closer to practical use

Quantum computers are expected to have profound impacts on society that extend far beyond the achievement of next-generation networks. However, many challenges for widespread adoption remain. Continued advancements in this area will play a pivotal role in making quantum computers a reality in the near future.